Vibrational spectroscopy provides the means to non-destructively assess the molecular composition of biological samples, trace metabolic and functional processes, and identify disease. In general, vibrational spectroscopy encompasses two methods: Infrared (IR) spectroscopy and Raman scattering. IR spectroscopy describes the direct absorption of photons in the IR region of the spectrum that match the vibrational energy levels of a molecule; while Raman scattering can be described as inelastic scattering, where the energy lost by the incident photons excite the vibrational modes. Because IR spectroscopy suffers from poor spatial resolution (due to the longer wavelengths used), we focus on Raman techniques. In practice, spontaneous Raman scattering is very weak, and as a result it can be difficult to separate the unique molecular signatures from the overwhelming Rayleigh (elastic) scattered light, or background fluorescence. Coherent Raman scattering, including stimulated Raman scattering (SRS) and coherent anti-Stokes Raman scattering (CARS), are nonlinear alternatives that enhance the weak Raman signal by means of nonlinear excitation, enabling imaging speeds up to video-rate [1-3].
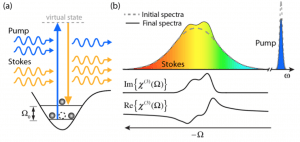
These methods have shown tremendous potential for advancing many areas of biology and medicine. However, due to the different sources of noise and technical constraints, SRS and CARS are currently limited to point scanning with (a) limited capabilities for spectral multiplexing (for SRS) [2,3], or (b) undesirable artifacts/background signals (for CARS) [4,5]. Recently, we have developed a novel approach based on SRS, SOCT and nonlinear dispersion to overcome such limitations [6-7]. The combined approach, termed SRS-SOCT, enables simultaneously multiplexed spatial and spectral imaging with sensitivity to many biochemical species that play an important role in biology and medicine. Fast, wide-area and volumetric molecular imaging, which can be accomplished with SRS-SOCT, would be extremely valuable for a number of clinical applications. The figure below shows a demonstration of the method.
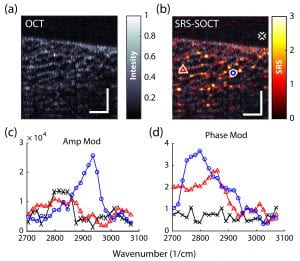
References:
[1] Camp, C. H., Jr, Camp, C. H., Cicerone, M. T., & Cicerone, M. T. (2015). Chemically sensitive bioimaging with coherent Raman scattering. Nature Photonics, 9(5), 295–305. http://doi.org/10.1038/nphoton.2015.60
[2] Freudiger, C. W., Min, W., Saar, B. G., Lu, S., Holtom, G. R., He, C., et al. (2008). Label-free biomedical imaging with high sensitivity by stimulated Raman scattering microscopy. Science Translational Medicine, 322(5909), 1857–1861. http://doi.org/10.1126/science.1165758
[3] Saar, B. G., Freudiger, C. W., Reichman, J., Stanley, C. M., Holtom, G. R., & Xie, X. S. (2010). Video-rate molecular imaging in vivo with stimulated Raman scattering. Science Translational Medicine, 330(6009), 1368–1370. http://doi.org/10.1126/science.1197236
[4] Evans,C. L., and Xie,X. S., “Coherent anti-stokes Raman scattering microscopy: chemical imaging for biology and medicine,” Annu. Rev. Anal. Chem. (Palo Alto, Calif.) 1(1), 883–909 (2008).
[5] Ploetz, E., Laimgruber, S. , Berner, S. , Zinth,W., and Gilch, P., “Femtosecond stimulated Raman microscopy,” Appl. Phys. B 87(3), 389–393 (2007).
[6] Robles, F. E., Fischer, M. C., & Warren, W. S. (2016). Dispersion-based stimulated Raman scattering spectroscopy, holography, and optical coherence tomography. Optics Express, 24(1), 485–14. http://doi.org/10.1364/OE.24.000485
[7]F.E. Robles, K.C. Zhou, M.C. Fischer, W.S. Warren, “Stimulated Raman Scattering spectroscopic optical coherence tomography,” Optica, 4 (3), 2017